Exploiting groundwater for low-carbon heating and cooling
MARTIN PREESE discusses the benefits of using groundwater to heat and cool buildings to deliver sustainable advantages that will reduce carbon emissions and stave off spiralling energy costs.Designers and developers of new-build and refurbishment projects for commercial and residential use are increasingly aware of the need to adopt the principles of sustainability in their work. Buildings are responsible for around half of the world’s generation of carbon dioxide. It follows that one of the primary objectives in the drive for more sustainable buildings should be to reduce the carbon emissions of a building through energy-efficiency measures and the use of renewable or low- and zero-carbon (LZC) energy systems. Specific targets for carbon emissions from buildings apply in the revised Part L of the Building Regulations. The use of renewable and LZC systems is likely to be increasingly considered by designers and developers to ensure compliance with Part L and meet the aspirations of customers and stakeholders. In the public perception, renewable-energy sources most commonly considered include those ‘from above’ such as solar or wind power. Technology in these fields is improving, but applications are constrained by climate and location. An alternative approach is to look for energy ‘from below’ — in the ground beneath the building.
Energy from the ground Traditionally, the way to obtain heat from the ground was via ‘true’ geothermal energy. This involves drilling boreholes down into specific types of rock which are kept very warm by the decay of radioactive minerals and extracting the warm water directly for heating use. The drawback with this approach is that it is dependent on the presence of ‘hot’ bedrock. This is comparatively rare, and suitable geological conditions exist in only a very few UK locations. This effectively prevents wide application of true geothermal sources. In the absence of geothermally ‘hot’ rocks, the temperature of soil and groundwater at moderate depth generally mimics the mean surface temperature. In the UK, between a few metres and, say, 100 m depth, temperatures are fairly constant all year round. Typical ground and groundwater temperatures are 10 to 14°C. These temperatures are too low to be used for direct heating. However, heat pump technology allows heat to be extracted from such low-temperature energy sources. Effectively the building is heated by chilling the ground beneath. Conversely, the building can be cooled in summer using a direct heat exchanger or the heat pump working in reverse to shed heat into the ground. Systems that extract or exchange energy with the soils, rocks or groundwater are generically known as ground-source systems. The two principal approaches used in ground-source systems are open loop and closed loop. Open-loop systems (Fig. 1) pump groundwater to the surface, where it passes through a heat transfer system, before being disposed of (at a different temperature) to waste or by re-injection back into the ground.
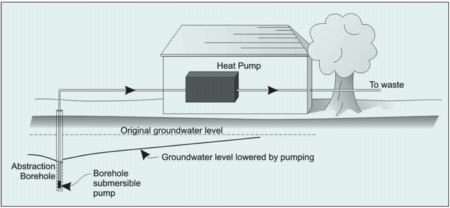
Fig. 1: Open loop ground source system
Closed-loop systems (Fig. 2) do not abstract groundwater, but instead circulate a fluid through a loop of pipes buried in the ground. The circulating fluid passes through a heat pump at the surface, and is then recirculated back through the buried closed loop to exchange heat with the ground.
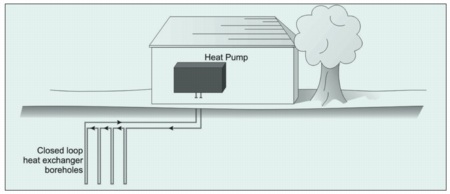
Fig. 2: Closed loop ground source system
Closed-loop systems generally influence a relatively limited volume of ground around the buried loop, and this tends to limit the peak heating or cooling loads that can be supported, even if multiple heat pumps are used. Most of the applications of closed-loop systems have had peak capacities of less than a few hundred kilowatts. In contrast, because open loop systems actively pump groundwater, they can draw water from great distances and can influence a much greater volume of ground, with a corresponding larger capacity to exchange heat with the ground. Some open-loop systems currently pumping from chalk strata in the UK have capacities in excess of 1 MW. Because of the potential for greater capacity, open-loop systems can be a realistic option for larger developments, when the economic capacity of closed-loop systems may be exceeded.
Groundwater as a source of LZC energy Strictly speaking, open-loop ground-source systems are not renewable energy systems. This is because they require electrical energy for water pumps and heat pumps, if used, to operate. Renewable sources such as wind, wave and solar do not require an external energy source. Nevertheless, open loop ground source systems are classed as LZC systems because they are very efficient, producing far more heating or cooling than the electrical energy they consume. An open ground-source system using heat pump can produce three to four more times energy as heating or cooling than it consumes in electrical energy. If a system can be run to provide cooling via a simple heat exchanger, without using a heat pump, efficiency is greatly increased, and the ratio of cooling energy delivered to electrical energy consumed can be in excess of 20. If a ground-source system is used to replace conventional heating (gas fired boilers) and/or air conditioning systems, utility costs (through reduced gas and electricity use) will be reduced. Even at 2005 gas prices, the cost savings were already worthwhile. In the future, gas prices are likely to continue climbing — making the substitution of gas-fired heating boilers with a ground-source system look attractive on variety of scales of projects. The lower operating costs of open-loop ground-source systems compared to traditional heating and cooling systems must be offset against the capital cost of installing the necessary boreholes, pipework, heat pumps and other equipment. Nevertheless, there is potential to reclaim the capital costs through operational savings within a relatively few years. Payback periods for commercial applications can be further reduced, by taking advantage of the Government’s Enhanced Capital Allowances for LZC technologies.
Not a panacea While open-loop ground-source systems can offer significant advantages, it must be recognised that there are two key constraints. The first constraint is the impact on groundwater resources. Open-loop systems can abstract significant volumes of water during a year (up to several hundred thousand cubic metres). Dependent on the nature of the strata from which the water is abstracted, such systems could adversely affect neighbouring abstractors or sensitive ecological sites such as wetlands by reducing the availability of groundwater. In England and Wales there is a legal requirement to obtain a licence from the Environment Agency (EA) to permit the abstraction; similar arrangements apply in Scotland from April 2006. As part of the licence application, there is a requirement to demonstrate that the environmental impacts arising from the abstraction are acceptable. If a licence is granted, it will set upper limits on the instantaneous flow rates and on the annual volume; these are likely to be key constraints for larger systems. If the EA considers that the abstraction for heating and cooling would have an unacceptable impact, a licence may be refused, so an open-loop system could not be implemented. The second major constraint is disposing of the water that has passed through the heat-transfer system. Cooling will produce water that is warmer than when it started, and heating will produce a waste stream of cooler water. Successful management of this stream of waste water is essential for the successful implementation of ground-water source systems. Where sites are near rivers or lakes, it may be possible, subject to obtaining a discharge consent, to dispose of the water to surface waters. However, the different temperature of the waste water in relation to the surface water may have an impact on the ecology on the surface waters. It is likely that the EA will set a limit on the temperature of water which can be discharged. In urban areas, where there is a sewerage network, it may be possible to discharge the waste water to sewer. If the waste-water utility can accept the water and its local hydraulic capacity is not exceeded, this can be a viable approach. However, the disposal charges payable to the wastewater utility may significantly affect the economics of the system. One approach is to re-injecting the waste water back into the soil or rock strata from where it came, via another set of boreholes. This approach has the attraction of avoiding surface water impacts and the cost of sewer disposal. It may also be easier to obtain an abstraction licence, as the net abstraction of groundwater is minimised or eliminated. However, the disadvantage of this approach is that, if the extraction and re-injection wells are not widely separated (perhaps by several hundred metres apart) warmer or colder water may ultimately circulate between the extraction and injection boreholes (Fig. 3). If this circulation causes the temperature of the abstracted water to vary by more than a few Kelvins, the efficiency of the heating or cooling system could be seriously reduced. Such a situation may take several years to develop, so there is a need for predictive modelling of the long-term interaction between the ground and such extraction and re-injection systems. Integration of predictive groundwater modelling into the design process is one of the immediate challenges, to avoid such systems failing early.
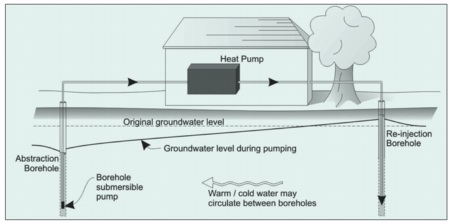
Fig. 3:Re-injection of water from heating and cooling systems
Conclusion Using groundwater as an LZC source of energy for heating and cooling buildings has real potential to reduce carbon emissions. There are also operational cost savings to be obtained, and, as we are seeing right now, future increases in gas prices will increase the economic advantages. that can be obtained However, the future application of these systems may be constrained by two factors • The willingness of the environmental regulators to grant groundwater abstraction licences for cooling systems. If it cannot be demonstrated that such systems will have minimal impact on nearby abstractors and on the wider groundwater environment, designers and developers may find their applications for licences to abstract groundwater are refused. • The need to dispose of significant volumes of warmer or cooler water produced by the heat exchange system. One option that initially appears attractive is to dispose of the waste water by re-injection into the aquifer. However, this approach carries the risk of warmer/colder water migrating between the abstraction and re-injection boreholes, affecting the long term efficiency of the system. The immediate challenge for the designers and installers of groundwater source systems is to integrate predictive modelling of abstraction and re-injection of water into the design process. If this is not done effectively, there is a danger that clients may be become disillusioned if abstraction licences are not granted or long-term system efficiencies are not great as predicted from theory. If that occurs, the full potential of the environmental and economic benefits of groundwater source heating and cooling may not be realised. Consequently, and to avoid the pitfalls, it pays dividends to consult with an expert who can help to find the best and most cost-effective solution to using ground water to heat and cool buildings.
Martin Preene is UK groundwater manager with Golder Associates (UK) Ltd.
Download:
Related links:
Related articles: